Global warming has been one of the key risk the world is facing and most of the countries have committed to address it by reducing carbon footprint. In the construction industry carbon is mainly consumed at construction stage and at operational stage. Over the years operational carbon has been addressed by innovations and upgrades, construction stage carbon i.e. embodied carbon has not seen much reduction. In this paper the authors elaborate the embodied carbon concept, complexity in quantification, influencing parameters and explain how to measure, mitigate and benchmark this key parameter from structural engineering perspective and provide guidance to optimize.
Challenges in Evaluation and Benchmarking of Embodied Carbon of Buildings in India
Abhijeet Kulkarni, Anirudha Deshingkar and Aditi Kulkarni
Abstract
This paper was published in the Jan-Mar 2023 issue (Vol 13, No. 1) of Structural Engineering Digest (SED) published by the Indian Association of Structural Engineers (IASE). This is being re-published in LCCF Newsletter for wider dissemination with due permissions, both from SED-IASE and also from the authors.
Introduction
The world now is in a climate emergency. The landmark 2018 special report from the UN Intergovernmental Panel on Climate Change (IPCC 2018 report), Global Warming of 1.5ºC, presented a grim picture of the catastrophic world we will inhabit if global average temperatures rise by 2ºC compared to a 1.5ºC scenario [1]. The effects will be severe and, in some cases, permanent. This urgency demands that current unsustainable consumption models be drastically changed right away.
The 17 Sustainable Development Goals (SDGs) established by the United Nations General Assembly in 2015 as a part of the 2030 Agenda for Sustainable Development paved a way for promoting sustainable development – a development that meets the needs of the present without compromising the ability of future generations to meet their own needs.
Climate change and its impacts, including rising temperatures, sea level rise, and extreme weather events, pose significant challenges to achieving the SDGs. The World Economic Forum’s latest 2023 report recognises Climate Change is one of the top risks in near as well as medium horizon having potential to erode great economic as well. Further, “Failure to mitigate climate change” and “Failure of climate change adaptation” hold potential for ‘polycrisis risk’ as volatility accelerates [2].
2. India’s Commitments
India has made several commitments towards mitigating devastating effects of climate change since 2016. Despite being a growing economy and one of the most populous countries in the world, India updated its climate commitments time-to-time, taking steeper targets each time at the same time making a steady progress towards decarbonisation. As per the Nationally Determined Commitments (NDCs), India now stands committed to reduce Emissions Intensity of its GDP by 45 percent by 2030. The updated NDC also represents the framework for India’s transition to cleaner energy for the period 2021-2030. India substantially augmented its mitigation commitments and came forward with the Panchamrita (“the five nectars for immortality” in Sanskrit) strategy with the following five-point agenda:
- Increase non-fossil electricity generation capacity to 500 GW by 2030.
- Meet 50 percent of its energy requirements from renewable energy by 2030.
- Reduce the total projected carbon emissions by one billion metric tons from now (presumably 2022) through 2030.
- Reduce the carbon intensity of its economy by at least 45 percent by 2030 compared to 2005 levels.
- Achieve the target of net zero by 2070.
- Achieve 50 percent cumulative electric power installed capacity from non-fossil fuel–based energy resources by 2030. This was a clarification of a target in the COP26 national statement that said India would meet 50 percent of its energy needs from renewable energy by 2030.
It re-emphasised its target to create an additional carbon sink of 2.5–3 billion metric tons of CO2 equivalent by 2030. India has also ramped up its non-fossil electricity generation capacity since 2016, especially of renewables led by solar. Renewables now comprise an impressive three-quarters of the increase in total electricity generation capacity since 2016, with solar accounting for more than half of the total.
These commitments have already put India on accelerated path to not only decarbonise the grid (considering the coal has lion’s share in India’s electricity production) but also towards the 2070 net zero goal.
3. Construction Industry – contribution and impact
Fossil fuels – coal, oil and gas – are by far the largest contributor to global climate change, accounting for over 75 per cent of global greenhouse gas emissions and nearly 90 per cent of all carbon dioxide emissions. These anthropogenic carbon dioxide emissions are the single biggest cause for raising global temperatures and accelerating climate change.
Built environment sector alone is responsible for 39% of annual global GHG emissions, with 28% of all emissions coming from building operations and 11% coming from building materials and construction; the built environment sector has a vital role to play in addressing the climate emergency [3].
According to the definition of the World Green Building Council (WGBC), embodied carbon is “Carbon emissions associated with materials and construction process throughout the whole life cycle of building or infrastructure”. These emissions occur throughout the whole life cycle of a building, as illustrated in Fig 1 .
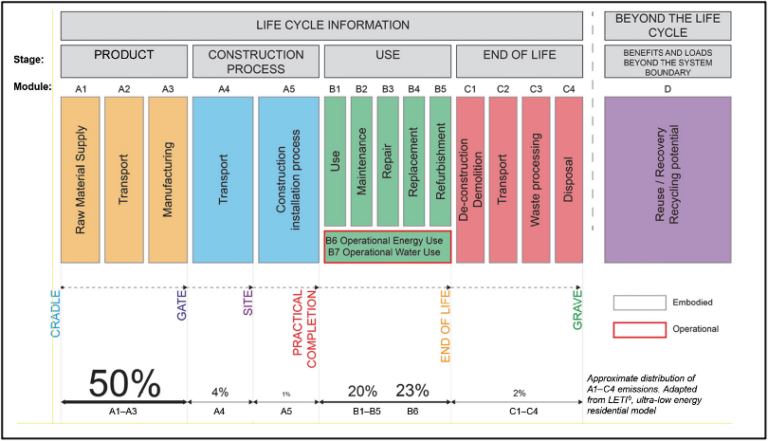
Fig 1 Stages of the whole life cycle of a building
Emissions occurred during the Product Stage and Construction Process Stage (A1 to A5) are generally categorized under the heading of “Embodied Carbon” whilst emissions in Use Stage (B1 to B5) are categorized under Operational Carbon. Although in operation and end of life stage carbon emissions also contribute to embodied carbon, however the overall percentage is significantly lower than those in A1-A5 stages.
The embodied carbon includes all carbon emitted during the following processes:
- manufacturing of material,
- transportation of material from manufacturing plant to assembly,
- storage and packaging,
- transportation of material to the site and storage at site,
- installation of the material at its final location,
- machinery and labour usage at site,
- construction stage waste.
4. Embodied carbon – IStructE methodology and calculations
Traditionally, efforts on reduction in carbon have focused on operational carbon and improvement in efficiencies of the products in operation. This has resulted in energy efficient products that enabled the industry to reduce the operational carbon to a large extent. Fig 2 shows how in-use CO2 has reduced from 1980 to 2030.
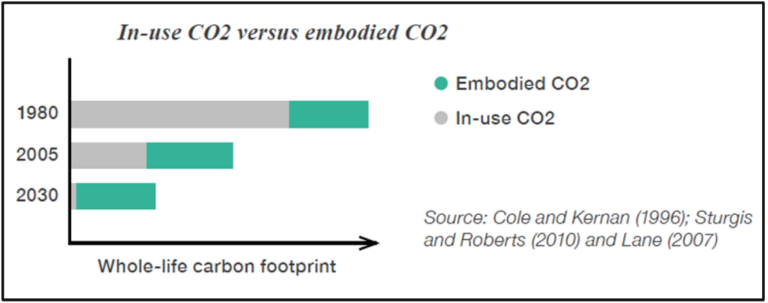
Fig 2 In-use CO2 versus embodied CO2
Recent studies have however shown that almost 50% of total carbon is produced while manufacturing of the materials (cradle to gate – A1 to A3) and further about 5% gets added during the construction process (A4-A5), Fig 1. Thus, in order to reduce the overall carbon footprint in the future, considerable efforts are needed in measuring, moderating and reducing the embodied carbon.
Definition of embodied carbon is developed and refined through years and most inclusive definition is provided in the guidance publication from Institution of Structural Engineers, UK (IStructE) “How to Calculate Embodied Carbon” [4]
According to IStructE, embodied carbon is the carbon emission associated with,
- Extraction and manufacturing of materials and products
- In-use maintenance and replacement
- End of life demolition, disassembly and disposal
Thus, embodied carbon is broadly classified as,
- Carbon emission from inception of material to practical completion in Modules A1 to A5, also known as ‘Upfront embodied carbon’
- Carbon over the life cycle associated with Modules B1 to B5 and Modules C1 to C4
Embodied carbon, therefore, will continue to increase in significance as a share of overall emissions even when operational carbon is reduced. While reducing emissions, operational carbon must remain a priority; there is also a pressing need to step up worldwide efforts to reduce embedded carbon emissions.
5. Buro Happold Approach
A ‘Net zero carbon building’ is the one where the amount of carbon emission during its life cycle is zero or negative. For structural engineering, ‘Upfront Embodied Carbon’ is important, and it contributes to almost 50% of the total life cycle carbon. The important first step in targeting ‘Net zero carbon building’ is, therefore, to measure current carbon embodiment for every stage of the project. The equation to calculate embodied carbon is very simple and can be presented as:
Emboided Carbon = Quantity as per design X Embodied Carbon Factor (ECF) per unit
The key term in the equation is Embodied Carbon Factor per unit which depends on many variables, chief amongst which include:
- Manufacturing processes emissions
- Transportation emission – depends on the vehicle conditions, road conditions, distance travelled, fuel used etc
- Construction stage emissions – depends on the machinery used, fuel used, waste control etc.
When talking about greenhouse gas emissions (GHG) emissions, it is a common practice to use the term "Carbon", as large portion of the emissions are from carbon-dioxide. However, there are other harmful gases such as methane (CH4) and nitrous oxide (N2O), etc which are responsible for GHG emissions. Therefore, the measurement unit for the carbon factor is always in terms of carbon-dioxide equivalent, i.e. kgCO2e/kg.
In European Countries, it is mandatory for the vendors to declare carbon factors for cradle to gate (A1-A3) modules. In the UK, this information is processed by the Inventory of Carbon and Energy (ICE) to develop standard values for the main material.
In India, however such information is not readily available for all the construction products. In 2017, International Finance Corporation (IFC) in consultation with a number of manufacturers, suppliers and developers published first draft of carbon factors applicable to Indian conditions. Although efforts have been made in the report to reach out as many stake holders as possible, the database is still a “work in progress” and would need to be used carefully.
Table 1 below provided generic comparison of the commonly used materials in structural engineering. Embodied carbon factors in the first row are from the IFC report generally applicable for Indian conditions whereas the second row shows carbon factors as per the ICE database generally used in the UK.

Table 1: Embodied carbon factors for concrete and steel
It is important to note that, due to the difference in the carbon factors, embodied carbon calculations in Indian commercial projects are generally 10 to 15% higher. However, it is also equally important to note that whilst calculation of the embodied carbon per project is critical, reduction of the same is the most important aspect. Thus, irrespective of which carbon factors are used, the project team needs to ensure that embodied carbon is getting reduced with respect the baselines during A1-A7 stages.
Benchmarking of embodied carbon is an ongoing process throughout the world. Benchmarks are dependent on multiple factors as discussed earlier and therefore it is still a “work in progress”. At Buro Happold, all new projects are evaluated during design stages and rated as per Table 2. Efforts are then made to improve the rating in the subsequent design stages and ultimately into the construction documentation.
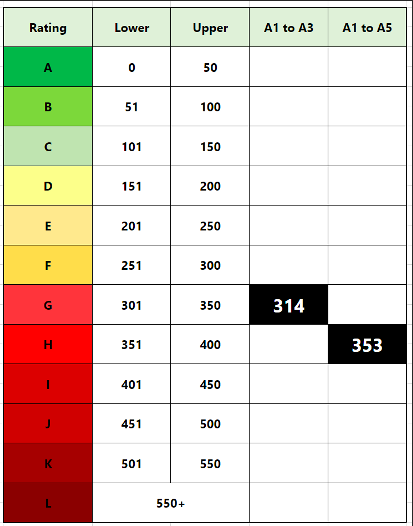
Table 2: Lower and upper rating limits followed by Buro Happold
Selection of the materials during construction and managing of the construction (machinery, labour, wastage etc) at site also plays vital role in overall reduction of embodied carbon. However, in Indian conditions, this is generally left with the contractors and the project management teams.
In-house developed tool for assessment of embodied carbon in structural elements, provides embodied carbon values distribution across various elements of a structure which helps in reviewing the elements requiring more focus to optimise the carbon embodiment in the project.
This approach of optimising carbon embodiment helps in reviewing design decisions, member sizing in a different perspective apart from focusing on construction time-line, rebar ratio and other similar performance indicators.
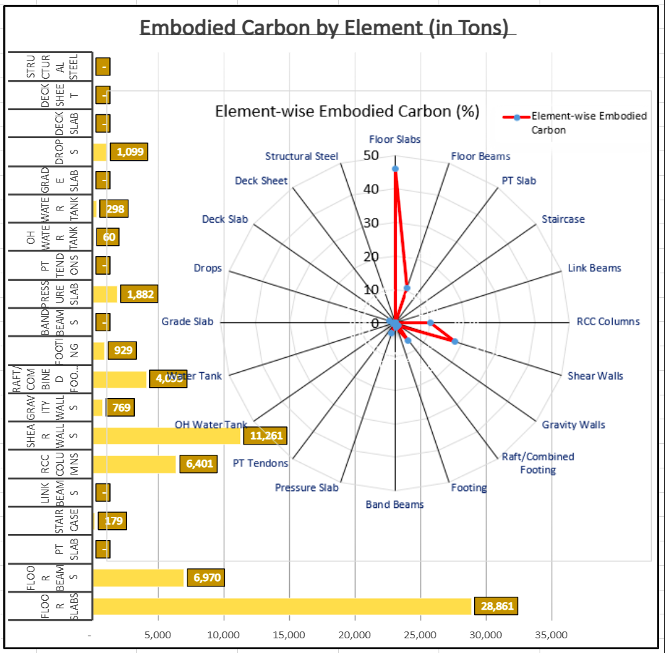
Fig 3 Element-wise embodied carbon in %
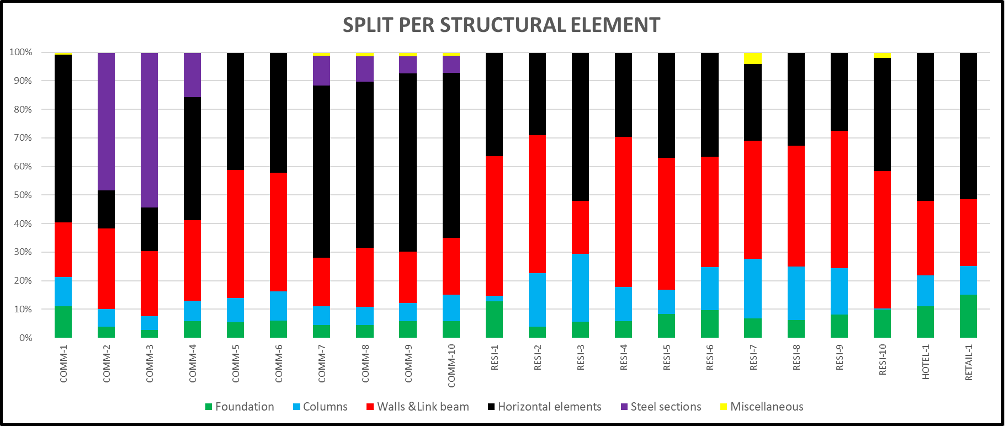
Fig 4 Structural element-wise splitting of embodied carbon values in different projects
Figs 3 and 4 present split of the embodied carbon in various elements of structure thus suggesting focus areas for each project for further optimization.
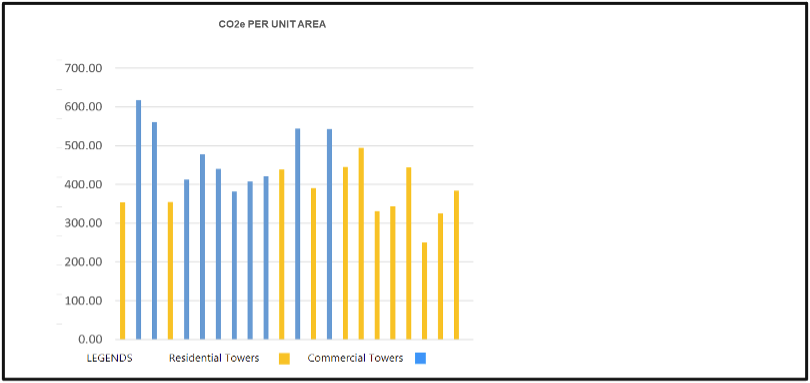
Fig 5 Carbon dioxide equivalent per unit area
Fig 5 shows, comparison of embodied carbon per unit area based on building usage. It has been observed that commercial tall buildings have higher emission per unit area than the residential tall buildings. This is mainly attributed to following factors:
- Long column-free spaces needing thicker floor plates
- Concentrated load carrying elements i.e perimeter columns and central core walls resulting higher floor-to-wall ratio
- Higher loads
- Use of higher-grade concrete needing increased cementious percentages
- Thicker foundations
- Increased number of below grade basement levels, etc.
6. Way to optimize and reduce
From the standpoint of GHG footprints, embodied carbon emissions can be allocated across Scope 1, Scope 2, and Scope 3 GHG emissions. It is important to understand who is directly responsible for these emissions in order to properly allocate it to different scopes.
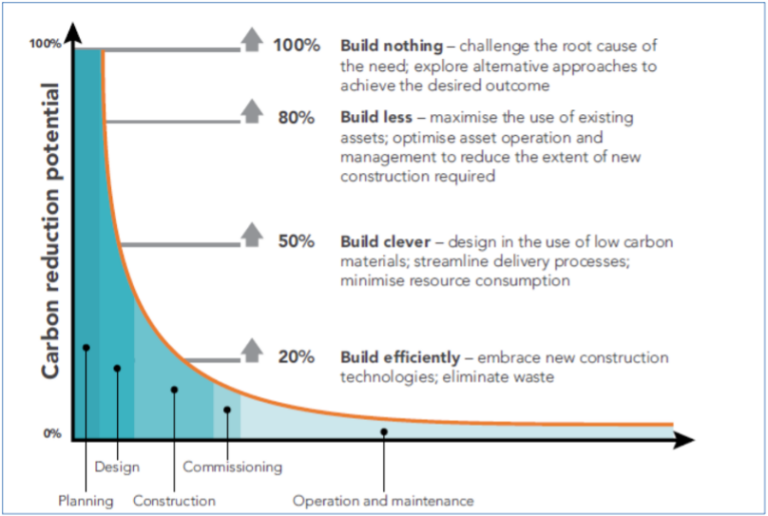
Fig 6 Carbon reduction opportunity (Source: Green Construction Board ICR, from UKGBC)
Embodied carbon is directly related to the materials used in building elements/layers. In Indian context, concrete and steel which are carbon-intensive materials, are used on a large scale for the superstructure and substructure of a building. In addition, cladding (finishes) is also responsible for a significant embodied carbon balance in a building.
Decisions needed to be taken at early stage of the design where opportunities for carbon reduction are evaluated that include challenging the design brief, loads and load combinations, code provisions, material usage and strength measurement criteria, etc.
Studies carried out at Buro Happold on carbon emission per m2 of area are shown in Fig 7 for different design alternatives. It can be seen that comparison of span arrangements versus type of material usages shows that, Timber construction provides least embodied carbon in European countries where timber in locally available and timber construction is suitable for the climatic conditions. In Indian conditions, PT Slabs with cement replacement using GGBS provides optimal embodied carbon:
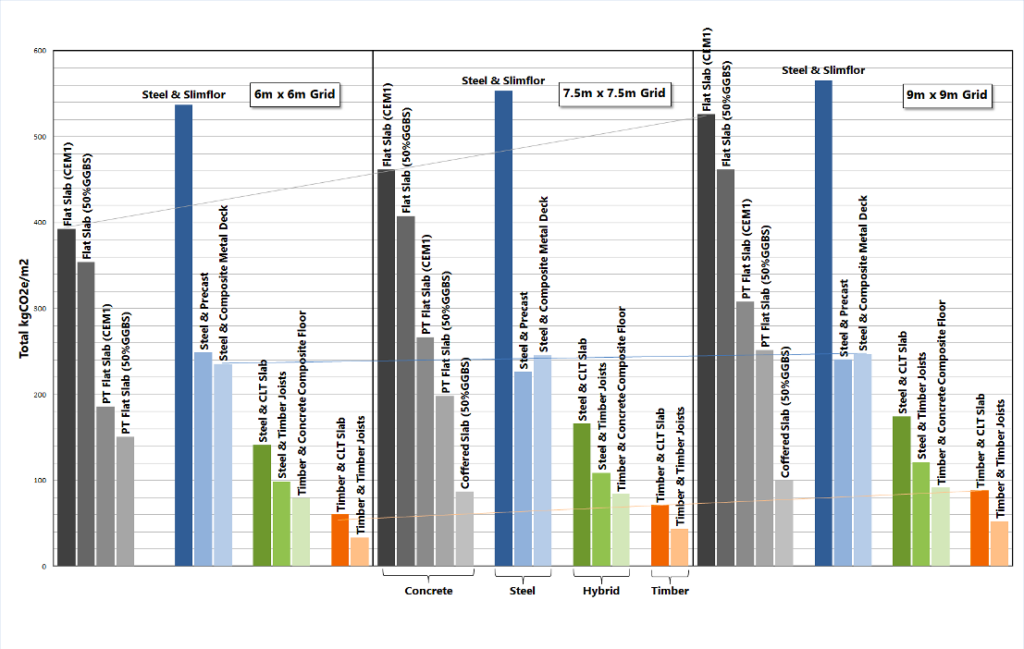
Fig 7 Carbon emission per m2 of area for different alternatives (Source:Buro Happold Inhouse Studies)
Key take aways from the studies done at Buro Happold are:
- The largest single factor affecting total CO2e is the GGBS content of the concrete, it can reduce CO2e by up to 45%.
- Waffle slabs are the most efficient form of RC slab but PT and slab on beam are also significantly better.
- M40 concrete is the most efficient grade, both reducing or increasing the grade increases the total CO2e.
- Increasing flat slab thickness and reducing the reinforcement quantity has an adverse effect on total CO2e.
- Minimum strength and cement content for blinding
- Work with civils on their mix design so as to optimize concrete mixes
- Avoid allowing upping cement content on site when programme slips
- For composite decks, the profile has a marginal effect on total CO2e
- Grade S460 helps to reduce CO2e but is less readily available – S355 is a reasonable compromise.
- Long span precast planks are significantly less efficient than using secondary beams to break up a span.
- Slimflor and UC beams have poor CO2e
- Understanding the carbon emissions associated with different durability and fire protection techniques
In conclusion, this means that setting reduction strategies and targets for these building elements will impact overall embodied carbon emissions. Examples would be:
- Building lighter (i.e. Review structural loads),
- Building wiser (ie. understand where the carbon hotspots are),
- Building low-carbon (i.e. alternatives from concrete and steel, avoid cladding when possible, etc.).
- Circular Economy – Reuse/Deconstruction
- Carbon Sequestering Materials
7. Challenges in Indian context
Major challenges in consideration of embodied carbon for overall GHG footprint reduction exercise are summarized in Fig 8. Key challenges are described below.
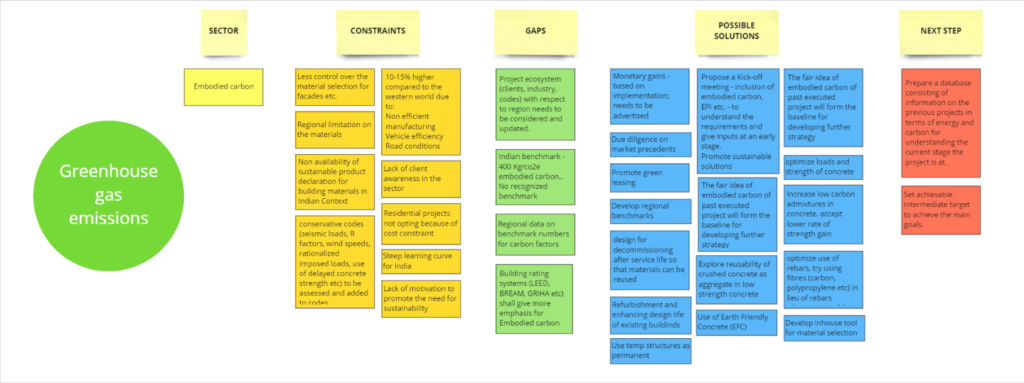
Fig 8 Challenges in reducing the embodied carbon : Constraints, gaps and possible solutions
- Currently there is no centralised database for the construction materials that provides values of embodied carbon factors.
- Further, there is no legislation that requires manufacturers to publish this data which may be readily available for sustainability practitioners. In absence of product specific data, it is rather an uphill task to benchmark embodied carbon for a particular project.
- In addition to this, globally there is not much information available on the façade materials and their product carbon foot print.
- There is no incentivisation from the regulatory authorities if a developer opts for low embodied carbon structure. Even with the most evolved green building certification regime (LEED- Platinum), there is very low weightage given for the projects which disclose embodied carbon.
- Although efforts are already made to improve and modernize codes and standards, it is still work in progress and would need to continue to bring the knowhow to international level that includes material and load factor rationalization, upgrade to seismic and wind codes, studies on seismic contour maps (similar to IBC) and optimisation of basic wind speeds, optimisation of imposed loads etc.
- Collective development of a framework to make it mandatory for civil engineering products and projects to declare embodied carbon values to bring more uniformity.
- Therefore, regulations and rating schemes, providing incentives to project teams that demonstrate increased use of products with EPDs will help in better disclosures on embodied carbon. Product manufacturers thereby realizing the competitive advantage can make significant increase in the number of available EPDs over the years.
References
- Intergovernmental Panel on Climate Change (IPCC) Global Warming of 1.50C Summery for Policy Makers, October 2018, IPCC, Switzerland.
- World Economic Forum, The Global Risk Report 2023, 18th Edition, Source: https://www3.weforum.org/docs/WEF_Global_Risks_Report_2023.pdf
- The Global Alliance of Building & Construction in their Status Report-2020, https://globalabc.org/sites/default/files/inline-files/2020%20Buildings%20GSR_FULL%20REPORT.pdf
- Institution of Structural Engineers (IStructE), How to Calculate Embodied Carbon , First published August 2020, U.K.
Authors

Abhijeet Kulkarni
Abhijeet Kulkarni is a Partner and Country Director-Structures at Buro Happold India. He has over 28 years of experience in consulting Civil and Structural Engineering field delivering tall, large and complex projects in the Middle East (UAE, KSA, Qatar), India, Kazakhstan and Libya. He has spent over 15 years of his career in the Middle East until mid-2019, where he was heading structural engineering team of leading European consulting firm. He has worked on projects ranging from high rise towers and special structures to structural forensics and industrial buildings. Abhijeet has been instrumental is setting the embodied carbon agenda for Buro Happold (BH) projects in India. Abhijeet holds a Master’s in Engineering (ME) in Structural Engineering from VJTI Mumbai and is a Chartered Engineer and Fellow of IStrcutE (UK), Fellow with ICE (UK), Fellow with IEI and Fellow with IAStructE India.

Anirudha Deshingkar
Anirudha Deshingkar works as Associate Director – Structures at Buro Happold India. He has over 28 years of professional experience in Commercial and industrial Building, Marine Structures and Bridges. He has successfully handled more than 500 projects of different scales as a consultant to the client and a consultant for the contractor for ‘Design & Build ‘projects having 3600 view of project execution. He has been actively involved in structural assessment projects that covers aspects such as due diligence, condition survey, testing, redefining material parameters, assess structure capacity for revised loading and preparing report with recommendations. His international experience, knowledge of software compliments, his local knowledge of the industry always make him inquisitive and explore new challenges. Anirudha holds a Master in Engineering (ME) in Structural Engineering from College of Engineering Pune.

Aditi Kulkarni
Aditi Kulkarniis Associate Director – Sustainability at Buro Happold India. In her career spread over 18 years, Aditi has spearheaded multiple projects focusing on carbon (GHG) accounting and reduction strategies, environment, social and governance (ESG) policies, climate change risk mitigation (TCFD), green financing, and non-financial disclosures. This includes sustainability reporting in-line with standards recommended by global agencies such as International Integrated Reporting Council (IIRC), CDP and Global Reporting Initiative (GRI). Aditi holds a Master of Technology (M.Tech) in environmental science and engineering from the Indian Institute of Technology (IIT) in Mumbai.